Lasers enable internet backbone via satellite
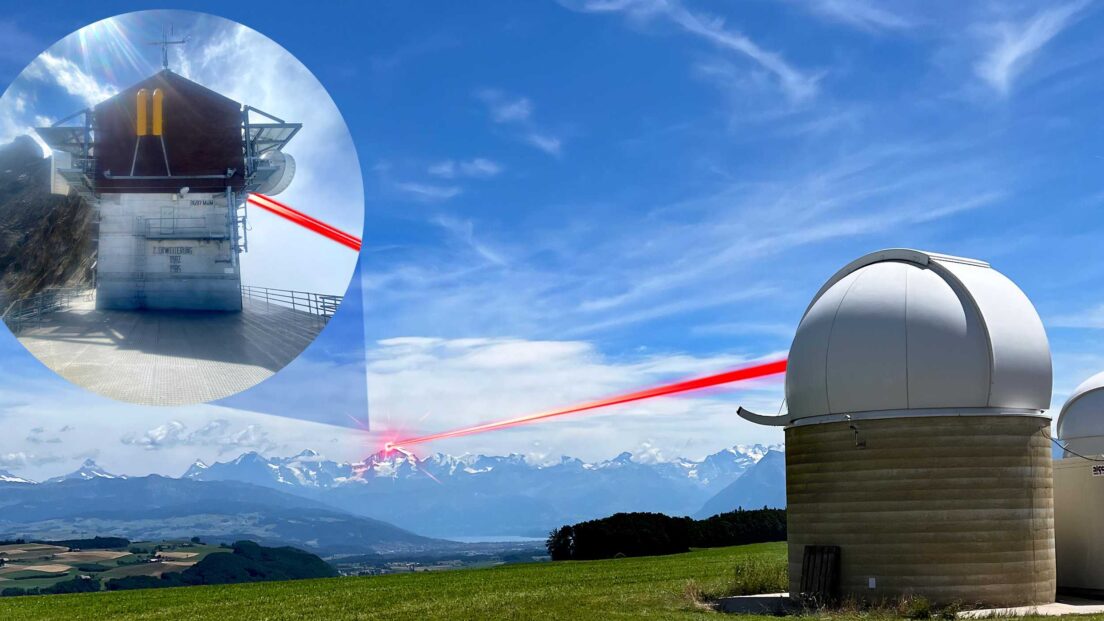
Optical data communication lasers can transmit several dozen terabits per second despite many disturbing air turbulences. This has been demonstrated by scientists from ETH Zurich together with European partners between the Jungfraujoch and Bern. This should soon make the costly construction of deep-sea cables unnecessary.
The backbone of the internet – the so-called backbone – is formed by a dense network of fibre optic cables, each of which transports up to more than a hundred terabits of data per second (1 terabit = 1012 digital 1/0 signals) between the network nodes. The continents are connected through the deep sea – and that is enormously expensive: a single cable through the Atlantic requires investments of several hundred million dollars. The specialised consulting firm Telegeography currently counts 530 active submarine cables. And the trend is rising.
Soon, however, this expenditure should no longer be necessary. Scientists at ETH Zurich have demonstrated optical terabit data transmission through the air in a European Horizon 2020 project together with partners from the space industry. In future, this will make it possible to establish much cheaper and also much faster backbone connections via satellite constellations close to the earth.
Challenging conditions between Jungfraujoch and Bern
However, the project partners did not test their laser system with a satellite in orbit, but with a transmission over 53 kilometres from Jungfraujoch to Bern. “Our test distance between the High Alpine Research Station on the Jungfraujoch and the Zimmerwald Observatory of the University of Bern is much more demanding from the point of view of an optical data transmission than between a satellite and a ground station,” explains Yannik Horst, the lead author of the study and a researcher at ETH Zurich in the Institute for Electromagnetic Fields headed by Professor Jürg Leuthold.
The laser beam had to move all the way through the dense, near-ground atmosphere. In the process, the manifold turbulences of the air gases above the snow-covered high mountains, the water surface of Lake Thun, the densely built-up agglomeration of Thun and the Aare plain influenced the movement of the light waves and thus also the transmission of information. The extent to which this flickering of the air, triggered by thermal phenomena, disturbs the uniform movement of light can be seen by the naked eye on hot summer days.
Satellite internet uses slow microwave radio
Internet connections via satellites are nothing new. The best-known current example is Elon Musk’s Starlink constellation, which uses more than 2,000 satellites orbiting close to the earth to bring internet to almost every corner of the world. To transmit data between satellites and ground stations, however, radio technologies are used that are much less powerful. Like WLAN (Wireless Local Area Network) or mobile radio, they work in the microwave range of the frequency spectrum and thus with wavelengths of a few centimetres.
Optical laser systems, on the other hand, work in the near-infrared light range with wavelengths that are around 10,000 times shorter and only a few micrometres. As a result, they can also transport correspondingly more information per unit of time.
In order to obtain a sufficiently strong signal at the receiver over long distances, the parallelised light waves of the laser are sent through a telescope that can be several dozen centimetres in diameter. This wide beam of light must then be aimed as precisely as possible at a telescope at the receiver whose diameter is in the order of magnitude of the received light beam.
Turbulence cancels out the modulated signals
In order to achieve the highest possible data rates, the light wave of the laser is also modulated in such a way that a receiver can detect several distinguishable states per oscillation. This means that more than one bit of information can be transmitted per oscillation. In practice, different heights (amplitudes) and shifts of the phase angle of the light wave are used. Each combination of phase angle and amplitude height then defines a different information symbol. With a 4×4 scheme, 4 bits per oscillation can thus be transmitted and with an 8×8 scheme 6 bits.
The changing turbulence of the air particles now causes the light waves to travel at different speeds inside and at the edges of the light cone. In the detector of the receiving station, this causes the amplitudes and phase angles to add or subtract each other to false values.
Mirrors correct the wave phase 1500 times per second
To prevent these errors, the French project partner supplied a so-called MEMS chip (micro-electro-mechanical system) with a matrix of 97 movable mirrors. The mirror movements make it possible to correct the phase shift of the beam on its cutting surface along the currently measured gradient 1500 times per second.
This improvement was essential to achieve a bandwidth of 1 terabit per second over a distance of 53 kilometres, as Horst emphasises.
New, robust light modulation formats were also used in the project for the first time. They massively increase the sensitivity of the detection and thus enable high data rates even under the worst weather conditions or with low laser powers. This is achieved by cleverly coding the information bits to properties of the light wave such as amplitude, phase and polarisation. “With our new 4D-BPSK modulation format (Binary Phase-Shift Keying), an information bit can still be correctly recognised at the receiver even with a very small number of only about four light particles,” Horst explains.
Overall, the specific competences of three partners were necessary for the success of the project. The French aerospace company Thales Alenia Space masters centimetre-precise targeting with lasers over thousands of kilometres in space. Onera, another French aerospace research institute, has the expertise in MEMS-based adaptive optics, which has largely eliminated the effects of air flicker. And the most effective modulation of the signals, which is essential for a high data rate, is one of the specialities of Leuthold’s research group.
Easily expandable to 40 terabits per second
The results of the experiment, presented for the first time at the European Conference on Optical Communication (ECOC) in Basel, are causing a sensation worldwide, says Leuthold: “Our system represents a breakthrough. Until now, it was only possible to connect either large distances with small bandwidths of a few gigabits or short distances of a few metres with large bandwidths using free-space lasers”.
In addition, the performance of 1 terabit per second was achieved with a single wavelength. In a future practical application, the system can easily be scaled up to 40 channels and thus to 40 terabits per second using standard technologies.
Additional potential for the new modulation format
However, Leuthold and his team will no longer concern themselves with this. The practical implementation in a marketable product will be taken over by the industrial partners. However, the ETH scientists will continue to pursue one part of their work. In the future, the new modulation format they have developed should also increase bandwidths in other data transmission processes where the energy of the radiation can become a limiting factor.